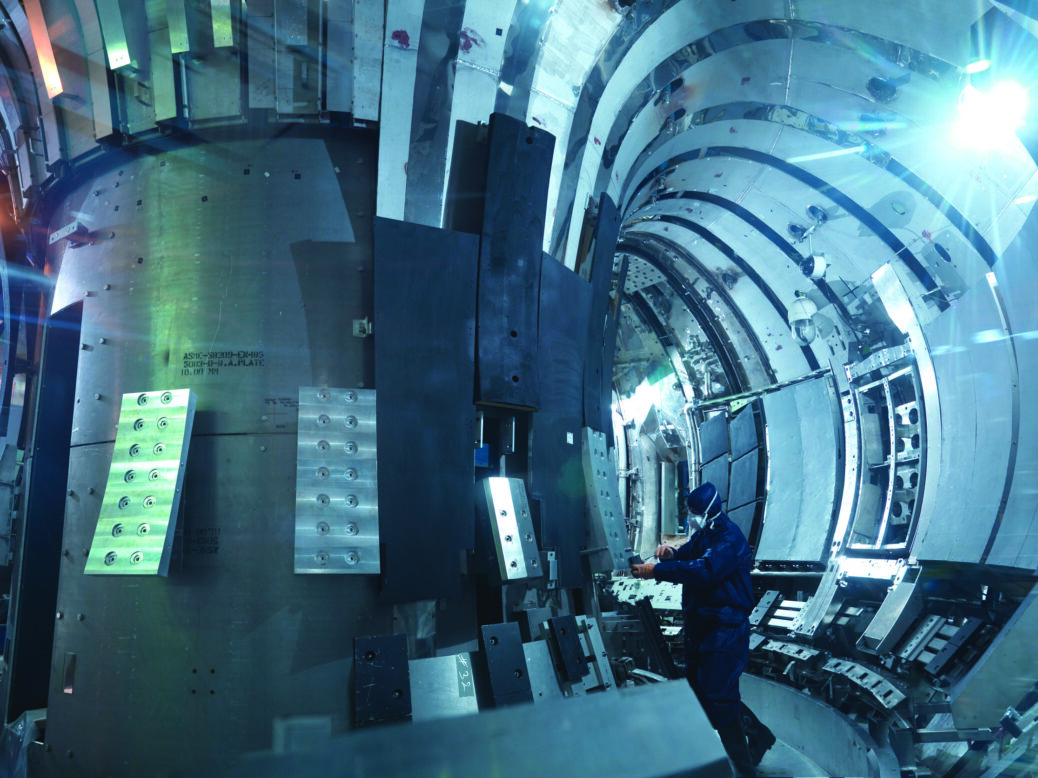
The Culham Centre for Fusion Energy in Oxfordshire is a set of large buildings with the unshowy, municipal appearance of a university or a hospital. But while hundreds of doctors – their PhDs mostly in physics, maths or engineering – walk the squeaking lino of its corridors, there are only two patients. Called MAST and JET, they appear as massive, roughly cylindrical assemblies of metal plates, wires and pipes. The larger of the two, JET, sits behind a pair of concrete doors that weigh 800 tonnes apiece. They are experimental reactors for testing nuclear fusion, the process by which stars release their energy.
Almost all human history has been powered by fusion, indirectly. Every plant that has ever been eaten, burned or fed to an animal used sunlight to make its energy, and all fossil fuels were once plants or animals. Fusion is arguably the universe’s most fundamental process. If it can be created and controlled on Earth, it will offer humanity an effectively limitless supply of clean energy.
For the last 50 years, the investigation of controlled fusion has been done almost exclusively in large, government-funded projects such as the Culham laboratory. There are good reasons for this; a working fusion power station is still a long way off, and the science is extremely difficult. For one thing, it requires a vessel that is capable of holding a substance hotter than the core of the Sun.
This substance is called plasma. There is no material that can survive contact with plasma heated to millions of degrees, so the JET and MAST reactors contain it with very powerful magnetic fields. Both are “tokamak” reactors – the word is shortened from a Russian phrase, and the design is Russian in origin – which means they hold plasma in a toroidal, or ring-shaped, magnetic field.
Tony Donné, the programme manager of Eurofusion, says that the research being done on the larger JET (Joint European Torus) reactor has two aims. “The first is to improve the plasma performance inside the machine” to make the fusion happening inside more powerful, and more controlled. “The second [problem] is the heat exhaust, which is one of the larger problems in fusion.” Even after cooling within the reactor, Donné explains, the heat exhaust in a tokamak can be “of the order of 10MW per square metre; it’s roughly the power flux that you have at the surface of the Sun. So these materials here” – he gestures to a series of metal plates, inches thick – “you can lie them on the Sun”.
Lorne Horton, head of the JET Exploitation Unit, says that JET is “the only operating device in the world at this scale. We can get conditions in the plasma that are closer to where we need to be” – hot enough that more power comes out of the reactor than is put into it – “than anywhere else”. For the moment this is true, but as Culham’s scientists – of which there are around 1,500 working on fusion – branch out, a number of private companies are starting to speed up the race for the energy source of the future.
Great balls of plasma
In a warehouse a few miles from Culham, a smaller but still formidable reactor – it stands around 15 feet high and fills most of a large room – has been built by a company called Tokamak Energy.
“Innovation comes from diversity,” explains David Kingham, Tokamak Energy’s co-founder and executive vice chairman, “and if one approach is not challenged at all, progress is going to be slower than if there’s a bit of rivalry and challenge, collaboration and competition.”
Kingham says the company was born from a shared frustration with the slow pace of fusion development. In the 1980s Kingham’s co-founder at Tokamak, Alan Sykes, showed that in theory a “spherical tokamak” – a reactor in which the plasma is held in a ball-like shape, which Kingham describes as a “cored apple”, rather than a the doughnut-like ring of the standard toroidal reactor – could be a more efficient design. Sykes worked at Culham at the time, and when he could not raise the means for a fully funded experiment to prove his theory, Kingham says Sykes “ferreted around the laboratory, found spare parts, and built the START spherical tokamak.”
Sykes and Mikhail Gryaznevich, “an expert in the physics of small tokamaks” who was recruited from the former Soviet Union, spent years working on the START reactor, setting records for important factors in fusion research such as plasma pressure. “Plasma”, Kingham explains, “is a squashy, awkward sort of thing” that “wriggles” under pressure, but massive pressure is crucial to creating the conditions necessary for fusion.
As in the large reactors at the Culham laboratory, this pressure is exerted using huge electromagnets. Some of these magnets – copper bars the size of railway sleepers – can be seen on the sides of the latest Tokamak Energy reactor. But these magnets present an engineering problem, in that copper, while it is a very good conductor of electricity, does provide some resistance. After less than a second at the power needed for fusion, copper magnets begin to get very hot indeed.
In 2012, Kingham, Sykes and Gryaznevich realised they could build magnets from another material. “A new superconductor was just coming onto the market in usable quantities,” remembers Kingham. Producing a strip of light, silvery metal tape, he explains that a single strip can hold thousands of amps of current, “if you cool it to around 20 degrees above absolute zero”. In terms of superconductors, “high temperature” is still very cold.
These magnets are now the company’s “particular expertise”. The longest any large, government-funded tokamak has maintained plasma is six and a half minutes, but using superconducting magnets, Tokamak Energy was able to get its spherical reactor to hold plasma for 29 hours. “It wasn’t wonderful plasma physics,” Kingham concedes, “but it did demonstrate that the magnets worked”.
Kingham sees the smaller, spherical reactor as a more realistic proposition. At eight to ten metres across, Kingham says a spherical tokamak could produce 150MW of power; Ofgem estimates that 1MW is enough to power 2,000 homes, which would enable a “small” reactor to power a city the size of Plymouth or Derby. He also thinks a reactor this size would be a better for the power grids of the future. “As increased amounts of wind and solar are added,” he explains, “you’ll need controllable power sources at the 100-megawatt, rather than gigawatt, level.”
“Plus, there’s the economics of it. These huge, Hinkley Point-scale devices are a huge expense, so they’re very challenging to get private finance into. Someone has to pay a premium, somewhere.” In the case of the Hinkley Point C power station, this someone is the consumer, who will pay the plant’s pre-agreed “strike price” for 35 years. The National Audit Office has estimated the extra cost of this policy at £50bn over the market price of the electricity.
While the government supports fusion research, Kingham says that “the publicly funded fusion projects think that they are in the 1960s, and someone has to get to the Moon… their view is that a lot more science needs to be done before it’s time for industry to get involved. We think fusion should be in the 2010s, and it should be like the space race is today. We’d like to see that transition to a more dynamic environment of innovation.”
The shrimp and the supernova
Though barely prawn-sized, at about 5cm long, the pistol shrimp is one of the loudest animals in the ocean. It has one large claw, half as long as its body, that it snaps at its prey, creating a shockwave that can stun or even kill small fish or other shrimp. In 2000, Dutch scientists discovered that the very loud snapping noise this creates – it is so loud that colonies of pistol shrimp can disrupt military sonar – was created not by the animal’s claw, but by what it does to the water around it.
When the shrimp’s claw moves through the water it creates cavities – bubbles of air and water vapour – in the liquid. At the same time, the moving part of the shrimp’s claw displaces a jet of water that smashes into the expanding bubble at more than 30 metres per second. This causes sudden compression of the gas inside the bubble, creating a tiny spark of hot plasma. Within the cavity, though it is minuscule and lasts a thousandth of a second, the gas reaches more than 4,700°C.
For Nicholas Hawker, co-founder and CEO of First Light Fusion, the pistol shrimp is an inspiration. It is one of only two examples of “inertial confinement” known in nature. The other happens within a supernova, the explosion with which some stars end their lives. In both cases, he explains, “there’s nothing holding the plasma together – no big magnets, or external forces”. Instead they are “transient phenomena, these very high-pressure, high-temperature, high-density states of matter, and they’re held together for a very short amount of time, simply by their own inertia. Once assembled, it just takes a certain amount of time for it to disassemble.” First Light Fusion is Hawker’s attempt to make fusion happen in the same way – momentarily, in bubbles.
In layman’s terms, Hawker’s plan is that rather than creating a large chamber, like the JET reactor, in which hydrogen is held and heated to 200,000,0000°C, he will take a tiny bubble of hydrogen and, like the pistol shrimp, he will shoot it. Except, instead of a claw, he will use an enormous gun made of magnets.
“We separate the fusion process into two parts,” he explains. “One is the target, and the other is the driver. So our target is inspired by shock-driven cavity collapse, and then the way we want to cause that collapse to happen, and the way to create that shock wave in the target is with a high-velocity projectile.”
The “target” Hawker describes is a one-centimetre cube of plastic, a bit like a dice, that contains a bubble two or three millimetres across. The “projectile” is a one-centimetre disc of metal, a bit like a penny. Hawker’s machine will use a huge electrical pulse to launch the penny at the dice at 20-30 kilometres per second. When the penny hits the target, it “creates a high-pressure shockwave, and that drives the cavity inside the target to collapse”, even faster than the projectile was travelling. As in the bubble created by the shrimp, the contents of the bubble are compressed to a superheated plasma. Except this time the bubble is much hotter, and it contains deuterium gas. As the deuterium nuclei are forced together, they fuse, releasing a burst of neutrons that can be used to generate electricity.
This may sound like a tremendous effort to go through for a waft of subatomic particles, but the energy released from fusion is so great that it could, Hawker believes, represent a serious power source. By First Light’s calculations, “each target would produce the same amount of energy as a barrel of oil.” In a power station, a target would be loaded into a chamber every ten seconds and shot, generating enough electricity to power thousands of homes. “You are going to get through a lot of targets – but think of the engineering effort that goes into producing a barrel of oil, and that’s still economically worthwhile.”
“We think there are some really interesting advantages of doing this, what sounds like quite an esoteric, thing”, says Hawker. “We’ve dodged two of the biggest engineering challenges for the tokamak – the heat flux, and the neutron damage to the sensitive parts. It’s super simple in comparison to what you have to have for a tokamak.”
All the same, Hawker says he will leave building the power station itself to “established engineering firms, who have decades of experience in managing huge projects. We’re going to be the team tackling the hard physics – which is all in the target. Our business plan is that we would manufacture the targets on-site, for the operator of the plant.”
This approach offers what everyone working in fusion craves – speed. Because First Light’s engineering is simpler, Hawker thinks a “first-of-a-kind” reactor, one that generates more power than it consumes, could be ready in as little as 12-15 years. While Hawker admits the “physics risk” of First Light’s technology is greater – meaning the risk that fusion will be harder to reliably produce or control – it’s an approach that, if it does work, could be much quicker to develop into a new form of clean energy.
Back at the Culham laboratory, teams of scientists and engineers are running tests that will inform the design of a new internationally-funded reactor, ITER, now being built in France. More than physics papers, engineering solutions or even neutrinos, however, the Culham laboratory is producing something it has produced in great quality for half a century: people with the knowledge and the desire to bring clean power to the world. As these people move out into other facilities and businesses, they are gradually building something more than a reactor. “What’s interesting,” observes Nick Harker, “is that there are actually startups, whereas 15 years ago there weren’t. Now, there’s a community.”